| Problem | Literature review | Study description | Major findings | Applied questions | References
Problem
Interest in site-specific management of farm fields, often called precision farming, has grown rapidly throughout the agricultural community in the 1990s. With the development of technology to manage with precision, awareness of the degree and scale of variability has also grown. Toposequence variability - systematic or recurring variability along a slope from crest to depression - is very common. But, the magnitude of variability in a toposequence depends, in large part, on its scale, which in turn is a function of the landform.
Undulating to hummocky moraine, common on the Prairies, provides an excellent venue in which to study soil variability in toposequences of relatively short length (i.e. less than 100 m). The benchmark site at Provost in east-central Alberta furnishes such an opportunity (see Factsheet on the benchmark sites).
Literature Review
Spatial variability of soils in landscapes has been studied in terms of soil survey reliability2,3, soil development5 and erosional processes6,7. In the 1990s, such studies have mushroomed with the advent of site-specific management4. Methods for assessing spatial variability include use of transect techniques to sample toposequences9. The Provost benchmark site provided an opportunity to study soil variability along toposequences in a complex morainal landscape of the Prairie region8.
Study Description
The Provost benchmark site was established on cultivated land in 1990. Site characterization, sampling design, sample collection and preparation, and analytical methods have been described previously8. Data from a nearby parcel of uncultivated (native) land, studied in 1991 using the same methods, are also presented for some attributes. The two sites provide an interesting retrospective comparison that spans nearly 80 years of cultivation.
Several toposequences in each site were sampled using transects9. The "average toposequence" was 65 m in length at the cultivated site and 80 m at the native site. Topsoil samples were collected at each sampling point (10-m interval) in each transect. There were nine transects with 64 sampling points at the cultivated site, and seven transects with 61 sampling points at the native site. Attributes of the topsoil discussed in this factsheet are pH, organic C, total N, and plant-available K.
Major Findings
Dramatic differences in pH of the Ap horizon (plow layer) versus the native Ah horizon (topsoil enriched with organic matter) emphasize the impact of cultivation across the landscape (Figure 1). The pH of cultivated hilltops averages almost 2.5 units more than in lower slopes and depressions. In the native landscape, there is only about a 1.0 unit difference. Most hilltops (knolls) in the cultivated landscape, when in fallow, are clearly visible by their grayish colours in contrast to darker soils on adjacent slopes. Such knolls are dominated by calcareous soils. With carbonate contents of 1 to 11%, pH values averaging 7.5 are not surprising in such soils.
The grayish coloured hilltops of the cultivated site also identify the soils with the lowest organic C and total N contents in the landscape (Figure 2). Results from a related study showed that tillage dilution (mixing of topsoil with subsoil) and erosion have most affected the hilltop positions (crest and shoulder). There is roughly twice as much organic C and N on lower slopes and in depressions, where mineralization is the main process reducing soil organic matter.
C:N ratios, ranging from about 10:1 on hilltops to 11:1 on backslopes and footslopes, augment the carbon picture. The lower C:N ratios on the hilltops illustrate the role of plant residue. Return of crop residue to the soil has likely been the least on hilltops due to lower yields over the long term. In addition, fresh plant residue and light fraction organic matter have likely been more susceptible to erosion from hilltops.
The image of this variable landscape is sustained with the results for available K (Figure 3). Plant-available K levels are usually considered to be high in Alberta soils as illustrated by the native Ah horizons. While K levels are still moderate to high at the cultivated site, the significantly lower values across hilltops reflect dilution by underlying subsoil, and erosion of both topsoil and plant residues. Further, the highest levels of available K occur in the cultivated depressions, which implies deposition of K-enriched plant residues and soil eroded from surrounding slopes.
Applied Questions
How will big differences in pH across a field, as at the Provost site, affect crop management?
While high pH and the presence of carbonates are not by themselves detrimental to crop growth, interactions with nutrients like P may be problematic. In contrast, the acidic soils that dominate the footslope and depressional locations are borderline for optimum crop growth, and may become an issue if acidification worsens.
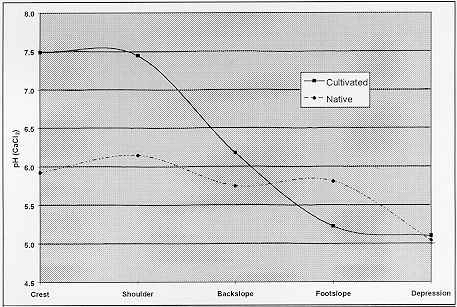
Figure 1 Variability in topsoil pH across average toposequences at the two Provost sites
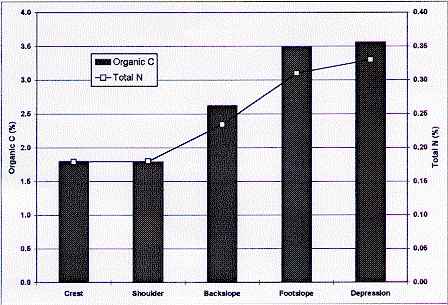
Figure 2 Organic C and total N contents of topsoil in an average toposequence at the cultivated site
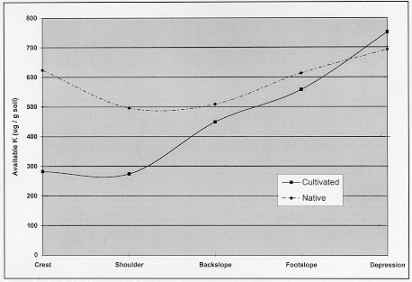
Figure 3. Available K content of the topsoil across average toposequences at the two Provost sites
More importantly, the wide range in pH at the cultivated site affects herbicide management. Herbicides belonging to the chemical family Sulfonylurea (e.g. Glean®) degrade slowly at pH 7.5 and higher, and can leave residues that are harmful to some crops for several years1. Also, the Sulfonylurea herbicides tend to be moderately mobile at high pH. In contrast, herbicides of the Imidazolinone family (e.g. Pursuit®) bind more readily to organic matter and clay below pH 6.5, decreasing their breakdown. In addition, anaerobic conditions, which occasionally occur when depressional areas are waterlogged, severely hamper degradation of Imidazolinone herbicides1.
Would expensive new equipment and computers be needed to do site-specific management in this landscape?
With its distinctive hilltops, some activities could be undertaken based only on visual assessments. For example, manuring of hilltops could be easily undertaken to increase carbon, improve soil structure and help reduce erosion. It would be more convenient, however, to use the latest electronic technology for managing some variables, in particular, for identifying and dealing with areas of low pH.
What other factors should be considered prior to developing a precision management system?
Questions about the best placement of inputs to optimize economic returns remain to be answered. A confounding issue is moisture. In this landscape, in this and drier regions of the Prairies, moisture overshadows many other variables. In dry years, crops on hilltops suffer; in wet years, crops in depressions may be drowned out while the hilltops provide near-optimum moisture. For any precision management system developed for this landscape, predictions about moisture supply must be made.
References
- Ahrens, William H. (ed.). 1994. Herbicide handbook. Seventh edition. Herbicide Handbook Committee, Weed Sc. Soc. Amer., Champaign, IL.
- Arnold, R.W. 1996. Soil survey reliability: minimizing the consumers' risk. Pages 13-20 in W.G. Nettleton, A.G. Hornsby, R.B. Brown, and T.L. Coleman (eds.), Data reliability and risk assessment in soil interpretations. SSSA Spec. Publ. 47, SSSA, Madison, WI.
- Arnold, R.W. and Wilding, L.P. 1991. The need to quantify spatial variability. Pages 1-8 in M.J. Mausbach and L.P. Wilding (eds.), Spatial variabilities of soils and landforms. SSSA Spec. Publ. 28, SSSA, Madison, WI.
- Bouma, J. 1995. Methods to characterize soil resource variability in space and time. Pages 201-207 in P.C. Probert, R.H. Rust, and W.E. Larson (eds.), Proceedings of - Site-specific management for agricultural systems - Second International Conference, March, 1994, Minneapolis, MN. ASA-CSSA-SSSA, Madison, WI.
- Donald, R.G., Anderson, D.W. and Stewart, J.W.B. 1993. The distribution of selected soil properties in relation to landscape morphology in forested Gray Luvisol soils. Can. J. Soil Sci. 73: 165-172.
- Gregorich, E.G. and Anderson, D.W. 1985. Effects of cultivation and erosion on soils of four toposequences in the Canadian prairies. Geoderma 36: 343-354.
- Kachanoski, R.G., Miller, M.H., Lobb, D.A., Gregorich, E.G. and Protz, R.D. 1992. Management of farm field variability I. Quantification of soil loss in complex topography. II. Soil erosion processes on shoulder slope landscape positions. Final Report No. 38. Technology Evaluation and Development Sub-Program, Soil and Water Environmental Enhancement Program. Agriculture Canada, Harrow, ON. 156 pp.
- Walker, B.D. and Wang, C. 1994. Benchmark site documentation: 05-AB (Provost, Alberta). Centre for Land and Biological Resources Research, Agriculture and Agri-Food Canada, Edmonton, AB. CLBRR Contrib. No. 95-03. 22 pp.
- Wang, C. 1982. Application of transect method to soil survey problems. Research Branch, Agriculture Canada, Ottawa, ON. Tech. Bull. 1984-4E: 34 pp.
Return to CAESA Soil Quality Program Research Factsheets
This information is provided by B. D. Walker, K. Haugen-Kozyra and C. Wang.
|
|